The future is fleshy...if we can actually grow it
A Q&A with Dr. James Henstock about bringing tissue-engineered products to market using mechanobiology
15 minute read
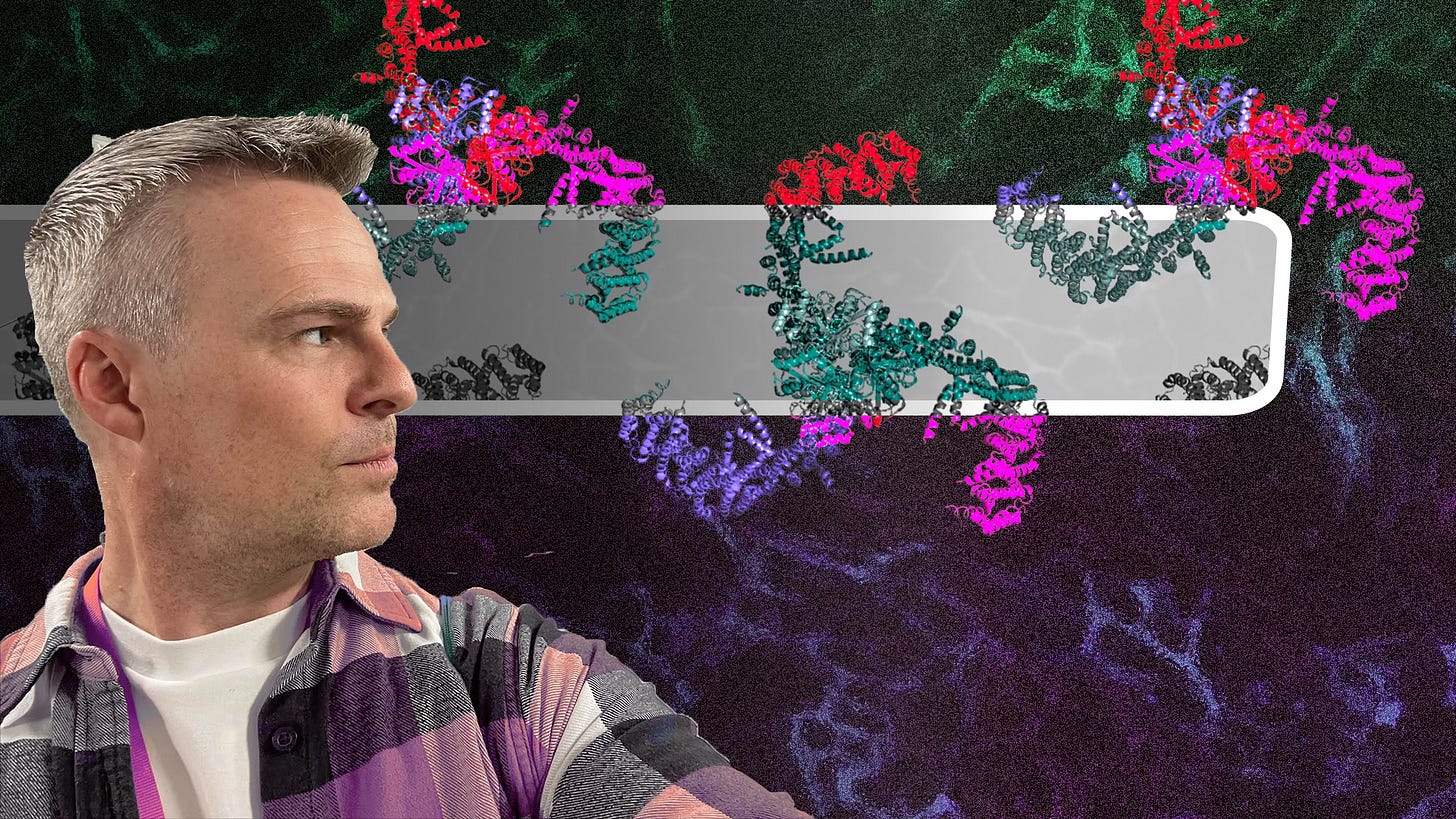
This week, I’m sharing a Q&A for those of you who want to dive deep into the particulars of mechanobiology (aka the study of how physical forces shape cell differentiation and tissue development). For those who want to read about the granular challenges involved in manufacturing and scaling tissue-engineered therapies within our current manufacturing infrastructure. For those who salivate over detail and who find themselves, like me, fascinated by people doing unconventional things.
Dr. James Henstock is one of those very people. Currently an Associate Professor in Bioengineering & Cellular Agriculture at Northumbria University, Henstock previously worked as a senior scientist at the cellular agriculture startup Vow, responsible for developing and scaling production strategies of Morsel, their “cultured umami quail [meat] product.”
Before joining Vow, Henstock was a tenure-track fellow and lecturer at the University of Liverpool following his postdoctoral research with Alicia El Haj during which, among other projects, he remotely stimulated the mechanotransduction pathways of mesenchymal stromal cells using magnetic nanoparticles to optimize bone tissue engineering.
At Northumbria, he currently works as part of the BioFutures working group, where investigators probe the idea of using biology to manufacture anything and everything. “Biology is really good at manufacturing things with scarce resources, so if you want to manufacture something complex and you don’t have much resource, use biology,” Henstock tells me.
From my last post we know bioreactors are enabling the study and engineering of complicated tissues. However, I left out an important point in that article. Bioreactors aren’t always very easy to use. They often leak. They can overheat. Maintaining contact between tissue and load cell can be tedious. And many research groups use custom-rigged systems that make it difficult to compare results between studies.
Bioreactors can also be inefficient. Most only allow you to run experiments on a small number of samples at once, resulting in studies that either take a significant amount of time to complete or finish with low n numbers. For Kyra Smith, PhD student in the Gottardi Biomaterials Lab, the sheer scale of physiologically-relevant tissue was her biggest challenge. “A tissue engineering construct for cartilage requires a minimum of 10 million cells per milliliter, and that is just so many cells to work with. It requires more planning and time to really prepare everything, and that's something not a lot of people, even within my lab who do engineering on different tissues, have to deal with.”
Theoretically, all those challenges can be designed out of systems for both lab- and industry-scale applications. And Dr. Henstock and I could have spent our entire interview discussing his body of research solving those problems, developing a versatile range of bioreactors for growing and testing tissues of all kinds. We could have discussed the fact that his lab prioritizes standardizing bioreactor design across the field, developing open-source, customizable, 3D-printable cell culture chambers that can be bolted onto any bioreactor system. We could have discussed his dedication to loading paradigms that diverge from traditional mechanical testing methods, reflected in the fact that he’s developed bioreactors that operate using hydrostatic pressure so tissue can be tested in more physiologically-relevant ways.
But we didn’t.
Instead, we talked about some of the grand challenges preventing mechanobiology from helping us manufacture tissue-engineered products, drawing on many of his experiences at Vow. I left our interview feeling optimistic about the future of the field, with renewed energy to break the mold and pursue more unconventional solutions to the challenges we face. I hope you walk away with some of that same spirit!
What we’re trying to do, growing tissues outside the body, is fundamentally unconventional. If conventional approaches haven’t been successful, why not try something that’s really unconventional?
The following interview has been edited for length and clarity.
Dr. James Henstock: I’m generally interested in science and everything that grows. Plants. Interesting animals. I think it’s all fascinating. There’s a whole load of things you can learn from biology that are outside what we normally study. Vertebrates, for example, have completely different ways of solving life’s challenges than we do for mammalian and human systems. I certainly think it’s worth learning about vertebrate biology to teach us about human biology.
It’s the same for engineering. I love doing woodwork. I love crafting stuff. I like making stuff. That was originally woodwork. I used to work with cars as well in my summer holidays between Uni. I used to work in a garage—my dad’s garage—working on cars. Those engineering-led hobbies really fed into what I was doing in terms of leveling up my technical skills for tissue engineering. There’s a lot we can learn from crafting.
The analogy I think is quite good is that back in the 18th and 19th centuries, all chemists were expert glass blowers because to do the organic chemistry they had to develop thrown organic chemistry kits. They were designing the apparatus and the hardware for the experiments they needed to do, and that was a really big period of innovation in organic chemistry. I think we’re going through a similar phase now that tissue engineering has matured. There is still a massive element of craft in tissue engineering. Making it more systematic naturally proves a challenge.
You can definitely tell some of the students approach [engineering] from a very technical perspective, and they produce tools which are functional but not beautiful. I certainly think you can tell when people actually care about both the form and the function and not just the function.
Matthew Fainor: And the form and function not just of our tools but also of our tissues, because all tissue has a specific function precisely because it takes a particular form, a beautiful pattern. When we think of engineers growing tissue in the lab, we are attempting to be patternmakers of sorts. Do you think we can ever engineer tissues like cartilage or tendon—do you think we can ever unlock those patterns—without the use of mechanically-loaded bioreactors?
No. Not at scale. If you want to seed a biomaterial with cells and implant it, fine. If you want to culture an authentic-replacement human tendon that actually has function, you only get that function through stretch, so you actually need to culture it for a period of time under stretch. And the same thing for cartilage. The extra element of cartilage is that it is thicker, so it requires the compression to help perfuse it. Biomechanics is the key to maturing tissues.
Why do you think we have a deficit of mechanobiology tools in industry? What are the challenges in bringing these tools to industry spaces? Is it the expense? The complexity?
Part of it is because there’s very little coherent information as to what stress would work [to grow functional tissue]. People know biomechanics is important, but if you’re going to invest in an extra level of manufacturing for a healthcare product, you have to have a very clear value-add benefit for that.
One of the areas I work in particularly is how biomechanics helps drive differentiation of things like mesenchymal stromal cells. If you could drive differentiation with mechanics, with a machine, instead of an input of a very expensive cocktail of growth factors, that could be a way we can justify having mechanobiology as part of the manufacturing process.
It all comes down to the fact that there’s not that many companies making mechanobiology rigs anymore. Ten years ago that wasn’t the case. Big companies like Instron, for example, saw there was a big desire for tissue engineers to look at mechanobiology. So Instron switched a large part of their company to start manufacturing mechanobiology bioreactors that fit into their load cells and mechanical testing rigs [in 2013 and 2014]. That was a large part of their business, and I don’t think it is anymore. I think they’ve sold elements of other companies, and those companies have been bought out by other companies. We worked initially with a company called Tissue Growth Technologies, and they sold up to Instron [in 2013]. And then Instron then sold that to a company in India who now don’t make the thing we designed. There’s been a loss in technology and a loss in market appreciation for bioreactors and mechanobiology.
If you could drive differentiation with mechanics, with a machine, instead of an input of a very expensive cocktail of growth factors, that could be a way we can justify having mechanobiology as part of the manufacturing process.
Do you think that’s because there are very few—I don’t know if there are any available products—that rely on a mechanobiologic solution to develop? Do you think it’s a self-fulfilling prophecy? Because we don’t have the mechanobiology tools to scale these products, we’re not going to develop the products. Because we don’t develop the products that may need these tools, we’re not going to make them because it doesn’t make sense to manufacture with tools we don’t have.
I think that’s definitely very true. It’s kind of mystifying. There are many physiological processes that rely on mechanical inputs. There’s probably a lot more research been done on cytokine signaling and growth factor signaling than mechanobiology. It’s easier to understand its pathways. One problem we have in mechanobiology, particularly in the last few years when mechanobiology has been a Nobel Prize-winning field and people have realized it has importance in cancer medicine as well, now every single pathway in the cell is mechanosensitive. It either has a mechanosensitive component or intersects with one of the traditional mechanosensitive pathways.
In contrast, you have Bone Morphogenetic Protein (BMP) signaling or Transforming Growth Factor-beta (TGF-beta) signaling, things that are very well characterized. When you put the growth factor on, you know primarily what pathway that growth factor is going to activate. If you hit the cells with a big force, it could be triggering 60 or 70 different pathways in unknown ways. And depending on the state of the cell at the time, you could have a very different response.
Understanding the pathways is a big problem to incorporating it into a standardized pipeline where you want to have a clear idea what your output—your cell output or your tissue output—is, based on the inputs you put in. And the regulators want clarity in each step. If you’re adding 10 micrograms of TGF-beta, you need to know what you’re going to get out at the end. If you’re adding a mechanical force and the cells will hopefully differentiate, that’s not quite as clear in terms of process. You want to be convinced you’re producing the right thing.
I think we’re missing a trick a little bit by not having an appropriate and consistent biomechanical environment when we give cells these different cocktails. In particularly with the work we’ve done with BMP, if you give the cells a subcritical dose of BMP that wouldn’t normally drive differentiation of stem cells into osteoblasts, but you do that in an environment that provides biomechanics, it actually amplifies the signal.
Not only do you get a more coordinated response, but from a biomanufacturing perspective, if you can use 10 times lower concentration of growth factors, that’s great for price point. It’s also great for regulators. It’s a bit of a win-win situation. There is a drive to do it still, but I think it’s hard to lock it into a process at this stage.
What are some of the barriers preventing discoveries like this from scaling, from being able to lock into the manufacturing processes?
A lot of cultivated meat, for example, has—in terms of the scalability—basically had to fit in with the types of bioreactors that were available to scale at that level. It’s been a challenge of having to fit the manufacturing process that we want into the hardware that was available. Cultivated meat is driving, with this new market, new revolution in tissue engineering and bioprocessing in terms of how you manufacture cells, how you generate a culture environment that you can mature those cells into something that looks like a tissue. I think that in the next three to five years, there’s going to be some big changes led by the cultivated meat industry that change everything we’ll be doing in tissue engineering.
There were three times in the first month when I was at [Vow], I said: “Don’t be ridiculous. That’s impossible.” And I was proved wrong. Three things that tissue engineering had told me for twenty years were impossible had been proved wrong in the first three months.
Do you think the cost-benefit of tissue engineering is ever going to put enough pressure on the industry to create feasible products, even with these new methods of biomanufacturing? What I hear from many clinicians in the field is “okay, it’s great that you’re working on this tissue-engineered technology, but if I can take a metal implant and put it a patient, they can see—potentially for the rest of their life—successful results. Why would I need a costly, potentially unpredictable tissue-engineered alternative?” Tissue engineers are focused on a small portion of the population that either needs these interventions early, experiences failure of traditional interventions, or requires interventions that restore multi-tissue complexes. I think it’s a hard sell, especially for cultivated meat. We’re putting money and resources into replicating animal tissue when there are cheaper alternatives on the table. Do you think that cost-benefit of tissue-engineered tech will ever reach scale?
I do. But I actually think cultivated meat is going to be an enabler for the rest of the field of tissue engineered products. I think, partly for reasons of driving down the cost of manufacturing and the scale at which you can make these things. But it sets out to subvert a lot of the established paradigms we’ve been working off in tissue engineering for a while. If we can introduce those shifts in perception back to tissue engineering, that will be quite transformative.
What your clinical colleagues have said is definitely true. I think maybe that’s where we’re going wrong with tissue engineering. We’re trying to recapitulate too much structure compared to a cheap alternative. By rethinking what the goal is, we should maybe, possibly in tissue engineering be implanting something that has a similar function, that helps healing but doesn’t completely recapitulate the biology. That could be the next phase in tissue engineering. Because trying to recreate a full intervertebral disc from scratch in the lab is always going to be really challenging. But do we need to? Can we create something that has an improved function over an artificial prosthesis?
What we’re trying to do, growing tissues outside the body, is fundamentally unconventional You’ve always got to be thinking—if conventional approaches haven’t been successful, why not try something that’s really unconventional?
In that spirit, what are you most excited about the work you’re currently doing?
The work I’m doing at the moment is trying to bring the technologies from cultivated meat back to tissue engineering. If we can solve scale up and economics, what can we produce in terms of tissue? The thing I’m most excited about at the moment is this project on antler biodesign, about how we can combine all the technologies that we know from cultivated meat and the manufacture of cells with everything that we know about tissue engineering to actually make structural quantities of bone. I find that really exciting because it throws a lot of the tissue engineering rulebook out the window. One of the things that’s held back bone tissue engineering has been porosity, as one example. You need a certain level of biomimetic porosity for it to be a useful bone implant for patients. Whereas you can say, “we don’t need that. We’re just going to make the pores as big as we need to get fluid through these [scaffolds].” By throwing out the rulebook and just doing slightly crazy things, I think we can learn a lot, and that’s certainly what really excites me about the work I’m doing at the moment.
The number of bone scaffolding porosity and pore size papers I’ve read in the last three years makes me never want to read another paper about them again. Truthfully, it feels like there’s so much information we’ve amassed and not a lot of actual outcomes into the hands of real people. And I don’t know what that disconnect is.
It’s almost like we’ve been trying to find the limits for the research—how we can limit ourselves. It’s such a large field of research. Focusing on what porosity size that we need helps define the experiments we need to do, but it also sets somewhat arbitrary boundaries on what you can achieve. Whereas the industry approach, particularly in cultivated meat, is to find the way around boundaries. There were three times in the first month when I was at [Vow], I said: “Don’t be ridiculous. That’s impossible.” And I was proved wrong. Three things that tissue engineering had told me for twenty years were impossible had been proved wrong in the first three months. That level of not being constrained and trying to innovate around a problem rather than seeing it as a hard edge, I think that’s what we need to do to be able to be really innovative in this tissue engineering space.